How does olfaction work?
The olfactory epithelium is exposed to the outside world and rests on top of the cribriform plate, the bone separating the nasal cavity from the brain. It includes a diverse niche of cells, including the olfactory sensory neurons (OSNs), sustentacular cells, basal stem cells, brush/microvillar cells and Bowman’s gland cells.
Olfactory sensory neurons, like the photoreceptors in the eye, are bipolar neurons, with one dendrite and one axon. Each OSN has only one type of receptor for one type of smell (odorant) alone. There is tremendous diversity in the epithelium for this reason, with millions of OSNs identifying over 10,000 different odorants, allowing the detection of a trillion smells. All the olfactory neurons, each transducing an odorant into a specific musical note, together create a harmonious, unique orchestra of a smell. It is the different combinations of odorants that makes something smell like gardenias or pizza.
Sustentacular cells (often referred to as ‘Sus’ in literature, unironically) provide metabolic and structural support to the OSNs. The olfactory epithelium is often referred to as ‘pseudostratified’ since the OSNs are not perfectly sandwiched in a single layer between these cells. These sustentacular cells are analogous in function to other cells in many tissues, such as astrocytes in the CNS; both have indirect, yet essential functions in homeostasis for the main player neurons to function effectively.
In between the cribriform plate and the olfactory epithelium is the lamina propria, which includes blood vessels, Bowman glands and nerve fibers. Capillaries here are lined with cells called pericytes, which are essential for blood brain barrier and blood pressure regulation.
Olfactory sensory neurons have their singular axons, together called the olfactory nerve, project across the cribriform plate into the brain. This path towards the olfactory bulb in the brain is lined by columnar-like organization of ensheathing cells, named that way for both their shielding organization around the OSN axon and their function to wrap the neuronal axons with insulating myelin sheath. These ensheathing cells are analogous to oligodendrocytes in the CNS and Schwann cells in the PNS. One may consider olfaction either an extension of the CNS or an exposed, PNS sensory organ, thus, these cells are an interesting breed.
In the brain, the axons of the OSNs synapse onto the olfactory bulb, specifically the glomerulus, which functions as the “thalamus” (sensory relay station) of smell. The glomerulus has cells called mitral and tufted cells which through postsynaptic receptors on their dendrites can receive and then project odorant signals to other regions of the brain. The axons of these cells together form the olfactory tract, which transmits the information to the olfactory cortex and other key components of the limbic system (involved in memory and emotions). Each synapse corresponds to a single note, or odorant. You can imagine that this makes sense evolutionarily since overlapping networks would complicate the communication of specific, diverse odorant information in the brain, whose function, like a computer, is to an extent binary, based on excitation or inhibition of action potentials.
Moreover, unlike the other senses, smell does not project/synapse onto the thalamus. Instead, the olfactory tract directly projects to the olfactory cortex to evolutionarily very rapidly detect odors that may be threatening or dangerous to survival; curiously, one does not need to be facing or directly in contact with something to smell so it is logical for it to have an adaptive role in survival. Even more, the direct, rapid connection of smell to higher order emotion and memory associated brain regions, such as the amygdala, has spilled over to our everyday vocabulary! For instance, the word ‘redolent’ linguistically first meant “strong smell” and now means “strongly reminiscent or suggestive of”. From a biological standpoint, it makes sense for people to sometimes associate a smell with a particular memory or emotion.
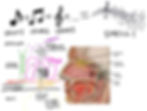
Spicy Mechanisms for Loss of Smell
While the exact mechanism for viral induced loss of smell remains unknown, there are several spicy possibilities.
The important thing to note is that loss of smell can happen at any point of the olfactory pathway. In order to think through the mechanisms of loss of smell once needs to think like a virus. This is why it is important to actually understand how olfaction works: so you can outsmart it. If you were the coronavirus, what would be ways you could tamper with the olfactory system? Well, you could….
Directly infect and cause lytic rupture of the olfactory sensory neurons (OSNs)
Cause physical congestion or obstruction of nasal pathway
Alter or damage the conformation/shape of the odorant receptor on OSN cilia that binds to the odorant so that there is anywhere from less affinity or no detection
Disrupt the signaling pathways/action potential downstream of the odorant binding its receptor
Infect supporting epithelial cells that signal and metabolically maintain the OSNs, so that the OSNs subsequently malfunction or die
Infect and tamper with the stem cells that regenerate OSNs and epithelial cells so that the epithelium cannot ‘bounce back’ to homeostasis as easily
Infect columnar ensheathing cells to reduce the shielding of olfactory sensory neurons axons as they project into the brain
Alter the blood flow to the area of infection by infecting pericytes in the lamina propria
Induce inflammation and suppress protective immune mechanisms to perturb the tissue and change gene expression profiles, affecting odor conduction. For example, proinflammatory cytokines may impede proper OSN function
Infect the downstream olfactory bulb as a way to prevent the signal from reaching the cortex and other higher-order brain processing centers
Multiply enough copies of yourself to increase chances of cell to cell transfer or endocytosis
Anosmia in COVID-19
Anosmia is a fancy term for “loss of smell”. It can either be complete or partial, but is usually temporary due to the regular cellular turnover in the epithelium. Anosmia can be due to physical obstruction in the nasal cavity or lesser understood mechanisms, such as inflammatory cytokine disruption of homeostasis. Interestingly, cases of anosmia in COVID-19 seem to appear in the absence of nasal obstruction, suggesting an alternative mechanism for loss of smell.
More than 70% of COVID-19 patients experience this temporary loss of smell, making it a good clinical biomarker. In fact, COVID-19 patients are 27 times more likely than healthy individuals to have loss of smell but only around 2 times more likely to have fever, cough, and respiratory difficulty. Studying SARS-CoV2 in the olfactory pathway could yield great insight of the mechanisms of coronaviruses in general.
As a quick review, the SARS-CoV2 virus is known to enter human cells through the ACE-2 receptor. Adjacent to ACE-2 is the protease (protein eating) TMPRSS2, which cleaves the S spike protein on the surface of the coronavirus so that it can then bind to the ACE-2 receptor in the correct conformation.
A study published in Science Advances found some interesting insights as to how CoV2 leads to loss of smell. The experimenters wished to know where in the olfactory epithelium the ACE-2 portal of entry is expressed so they could then speculate which cells the virus can infect in the first place. If there is no door for the virus to enter through, it stands to reason that it cannot infect those cells. Nevertheless this experiment rested on several limiting assumptions:
ACE-2 is the only receptor SARS-CoV2 can enter through: There is growing evidence of alternate methods of entry such as CD147, which may actually help explain the link of COVID-19 with blood clotting and more.
The threshold at which the experimenters detected ACE-2 expression is accurate: It is unknown how much ACE-2 expression is necessary for viral entry and infection; it could be low enough it may not have been detected in an assay.
The virus can only enter and infect cells if a receptor is present: There may be alternative mechanisms of entry, for instance, if the viral load reaches a large enough capacity in the extracellular space, it may be able to enter through endocytosis by pure chance.
The experimenters used bulk and single cell RNA sequencing to show the in vitro (in cells on a dish) and immunostaining to show the in vivo (in live animal) expression of the ACE-2 receptor. The findings revealed that ACE-2 and TMPRSS2 were surprisingly NOT detected in the olfactory sensory neurons. Nevertheless, they were detected in sustentacular cells, pericytes and stem cells of the epithelium. This suggests that the loss of smell was not due to a direct infection and lytic rupture of the OSNs, but instead due to an indirect, loss of the cells essential for their regeneration and regular maintenance. Moreover, in addition to loss of support there could be changes in the gene expression profiles of the cells proximal to the OSNs. The signals cells secrete to communicate with the OSNs may change and subsequently alter the transduction of the sensory information from the OSNs to the brain.
Based on this study, the presence of ACE-2 in olfactory epithelial cells poses a threat to both the homeostatic structure and function of the tissue (after all, structure and function are not mutually exclusive in biology). The notion of pericytes having high ACE-2 levels underscores the link of blood pressure to COVID-19 since ACE-2 is a part of the Renin-Angiotensin-Aldosterone system. Altered blood pressure maintenance due to the infection of these pericytes could alter the blood brain barrier and impact inflammatory responses. For instance, neutrophils, the first responder immune cells, squeeze across endothelial linings, where pericytes are, to tackle infection in epithelial tissue.
Nevertheless, despite the possibility that neuron loss is an indirect result of SARS-CoV2 infection, the study design’s assumptions remain limiting and other studies show inconsistencies that require further clarification. For instance, inconsistent with the proposed mechanism of the aforementioned study on ACE-2 expression in the olfactory epithelium, another study claims intranasal inoculation of SARS-CoV2 damages and infects both immature and mature OSNs of hamsters directly, not indirectly. This study localized the virus, instead of its receptor ACE-2, in the olfactory pathway in vivo. Regardless of ACE-2 expression, SARS-CoV2 infected the olfactory sensory neurons in addition to the other epithelial cells. This raises the eyebrow and the question: How did CoV2 sneak inside the neurons if they do not express the ACE-2 portal of entry? Nevertheless, the majority of the CoV2 infected cells profiled in this study were in fact not the neurons, suggesting that the discrepancy between the two studies could be due to a threshold problem: ACE-2 may be expressed at a low yet sufficient concentration for infection in the olfactory neurons such that ACE-2 expression was not detected in the first, described study.
What does seem consistent across the studies is that the olfactory bulb remains relatively ‘untouched’, with no ACE-2 receptor and no trace of CoV2. However, slightly more neuronal apoptosis in the bulb of COVID-19 mice than control, healthy mice suggests that the virus may have actually gotten that far into the brain or at the very least had some indirect influence through vascular pericytes or inflammation. Why and how the virus did not manage to spread to the bulbs if OSNs were infected upon inoculation remains a mystery.
All in all, the real answer to the question “Why is there loss of smell in COVID-19?” may lie at the intersection of these two studies on anosmia. It is likely that both the indirect effect of infected supporting tissue and vascular cells as well as the direct loss of sensory neurons are at the root of the pathology. In essence, this reflects the age-old trend for both primary infection and secondary inflammation playing a role, and often vicious cycle, in human infectious diseases.
Brainstorming Future Directions
Use of organoids or iPSC-derived human olfactory epithelium cell lines in vitro to better analyze the role of anosmia and COVID-19 in humans. The olfactory epithelium is not often studied or biopsied in autopsies routinely, so there is a dearth of data that leads scientists to depend on inexact animal models.
Investigate the role of blood brain barrier permeability and blood pressure regulation in COVID-19 neurological pathology and CoV2’s ability to enter the brain. As we discussed earlier, pericytes lining capillary vessels unsurprisingly express very high levels of ACE-2, leaving them potentially vulnerable to infection. How much of a role pericytes play in COVID-19 is unknown.
Evaluate the expression of alternate routes of entry of the virus in the olfactory epithelium, such as CD147.
Look into what factors are secreted by or what are differences in gene expression in olfactory epithelial cells under control and COVID-19 conditions using single cell RNAseq, for instance. This may help gain therapeutic insight into the factors that may lead to OSN malfunction or maintenance.
Repeat the study of intranasal inoculation of CoV2 in aging and young mouse models to see the impact of aging on the rate of infection, inflammation and tissue morphology. This may explain the more clinically severe vulnerability of aging human populations in COVID-19.
Better understand the link between anosmia, psychiatric disorders and Parkinson’s Disease. For instance there are dopaminergic neurons in the glomerular layer of olfactory bulb. These may play a role, despite not being the subpopulation of dopaminergic neurons that is most critically lost in Parkinson’s Disease.
Thank you for reading and please subscribe if you enjoyed :)
Follow @immunethought on Instagram for post updates!